A new family of molecules for self-assembly: The carboranes
To be useful in real-world applications, a self-assembled monolayer (SAM) of molecules on a surface must have a stable and controllable geometry. Researchers at Penn State and the Sigma-Aldrich company have found a way to control geometry and stability by making SAMs out of different carboranethiol isomers, which are cage-like molecules. The research results will be published in the March 2009 issue of the journal ACS Nano.
"Our results allow us to control the chemical and physical properties of the SAM without changing its structure," said team leader Penn State Distinguished Professor of Chemistry and Physics Paul Weiss. "The ability to control these types of properties enables us to create SAMs that, for example, selectively capture biomolecules from complex mixtures. These carboranethiol molecules give us exceptionally high-quality SAMs, largely because of their simplicity. This innovation opens up new capabilities in terms of patterning and control. We are trying to come up with simple and economical means to control the chemistry of a surface all the way from the wafer scale (several centimeters) down to the single-molecule scale (sub-nanometer)."
Using two different carboranethiol isomers, the team built SAMs that have identical geometries, as well as selectable interaction strengths. They found that they could easily control the chemical properties, including chemical resistance and wettabilitity, of the new SAMs. "The beauty of self-assembled monolayers is that with a one-nanometer or two-nanometer-thick film, we completely changed the chemical, physical, and biological properties of the surface," said Weiss.
To build the new SAMs, the researchers performed separate experiments in which they attached one of two, or both, versions of a carboranethiol molecule -- either a meta-9 isomer (M9) or a meta-1 isomer (M1) -- to gold surfaces through sulfur-gold bonds. Each of the carborane cages consists of 10 boron atoms and two carbon atoms. The carbon atoms have an unusual hexa-coordinated structure that gives them a positive charge. As a result, the carborane cages have a dipole moment -- a measure of the separated charge of a molecule that helps determine its interaction with water and other molecules -- that is either nearly parallel to the surface of the SAM (for M1, in which sulfur is attached to a carbon atom in the carborane cage) or nearly perpendicular to the surface of the SAM (for M9, in which sulfur is attached to a boron atom in the carborane cage).
"Both the M1 and M9 molecules form the same structure on the surface of the SAM, but the M1 molecules make the SAM more stable, while the M9 molecules make the SAM more hydrophilic, or wettable," said Weiss. "Each of these properties is beneficial for different applications."
Specifically, the group determined that the M1 molecules interacted more strongly with one another than the M9 molecules interacted with each other due to dipole interactions between the M1 cages. As a result, more energy was required to remove an M1 molecule from the SAM, thus creating a more stable SAM. On the other hand, the M9 SAM, although less stable, was able to become wet more easily than the M1 SAM because the dipole was oriented toward the outside of the SAM.
"Carborane chemistry is so well-understood that there is a lot we can do to exploit these SAMs," said Weiss. "For example, we can add more chemical groups onto selected spots on SAMs to determine the supra-molecular, or overall, properties of a SAM that go beyond the properties of a single molecule."
Weiss, his lab group, and Viktor Balema, a product manager at Sigma-Aldrich Co, plan to continue their work to develop a library of carboranethiols that can be used to make novel nanostructures and nanodevices. This work was supported by the National Science Foundation through its funding of the Center for Nanoscale Science at Penn State, by Penn State's National Nanotechnology Infrastructure Network, and by the Sigma-Aldrich Materials Science Initiative.
Topics
Organizations
Other news from the department science
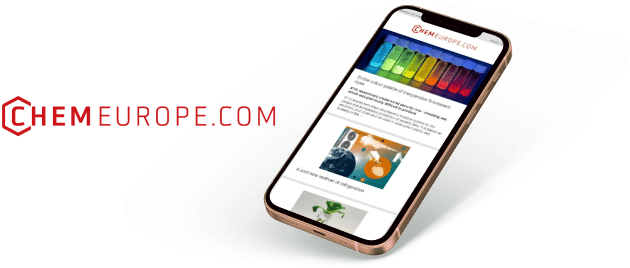
Get the chemical industry in your inbox
By submitting this form you agree that LUMITOS AG will send you the newsletter(s) selected above by email. Your data will not be passed on to third parties. Your data will be stored and processed in accordance with our data protection regulations. LUMITOS may contact you by email for the purpose of advertising or market and opinion surveys. You can revoke your consent at any time without giving reasons to LUMITOS AG, Ernst-Augustin-Str. 2, 12489 Berlin, Germany or by e-mail at revoke@lumitos.com with effect for the future. In addition, each email contains a link to unsubscribe from the corresponding newsletter.