More effective catalyst with light
Plasmonic effect allows catalyst to work at lower energy
Rice University nanoscientists have demonstrated a new catalyst that can convert ammonia into hydrogen fuel at ambient pressure using only light energy, mainly due to a plasmonic effect that makes the catalyst more efficient.
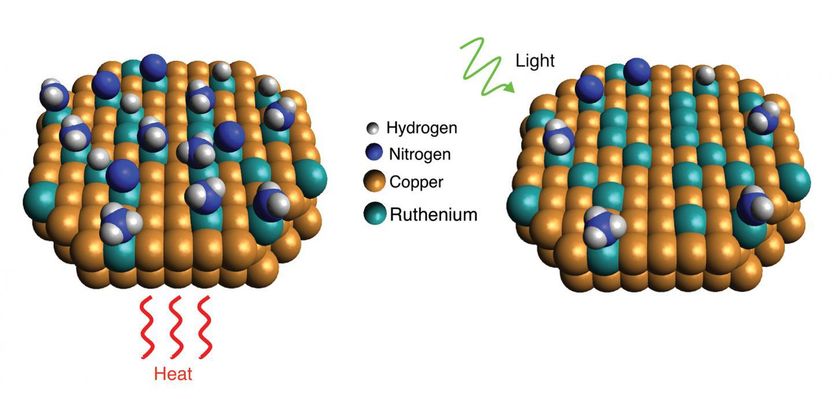
Scientists with Rice's Laboratory for Nanophotonics have shown how a light-driven plasmonic effect allows catalysts of copper and ruthenium to more efficiently break apart ammonia molecules, which each contain one nitrogen and three hydrogen atoms. When the catalyst is exposed to light (right), resonant plasmonic effects produce high-energy "hot carrier" electrons that become localized at ruthenium reaction sites and speed up desorption of nitrogen compared with reactions conducted in the dark with heat (left).
LANP/Rice University
A study from Rice's Laboratory for Nanophotonics (LANP) describes the new catalytic nanoparticles, which are made mostly of copper with trace amounts of ruthenium metal. Tests showed the catalyst benefited from a light-induced electronic process that significantly lowered the "activation barrier," or minimum energy needed, for the ruthenium to break apart ammonia molecules.
The research comes as governments and industry are investing billions of dollars to develop infrastructure and markets for carbon-free liquid ammonia fuel that will not contribute to greenhouse warming. But the researchers say the plasmonic effect could have implications beyond the "ammonia economy."
"A generalized approach for reducing catalytic activation barriers has implications for many sectors of the economy because catalysts are used in the manufacture of most commercially produced chemicals," said LANP Director Naomi Halas, a chemist and engineer who's spent more than 25 years pioneering the use of light-activated nanomaterials. "If other catalytic metals can be substituted for ruthenium in our synthesis, these plasmonic benefits could be applied to other chemical conversions, making them both more sustainable and less expensive."
Catalysts are materials that speed up chemical reactions without reacting themselves. An everyday example is the catalytic converter that reduces harmful emissions from a vehicle's exhaust. Chemical producers spend billions of dollars on catalysts each year, but most industrial catalysts work best at high temperature and high pressure. The decomposition of ammonia is a good example. Each molecule of ammonia contains one nitrogen and three hydrogen atoms. Ruthenium catalysts are widely used to break apart ammonia and produce hydrogen gas (H2), a fuel whose only byproduct is water, and nitrogen gas (N2), which makes up about 78 percent of Earth's atmosphere.
The process begins with the ammonia sticking, or adsorbing, to the ruthenium, and proceeds through a series of steps as the bonds in ammonia are broken one by one. The hydrogen and nitrogen atoms left behind grab a partner then leave, or desorb, from the ruthenium surface. This final step turns out to be the most critical, because the nitrogen has a strong affinity for the ruthenium and likes to stick around, which blocks the surface from attracting other ammonia molecules. To drive it away, more energy must be added to the system.
Graduate student Linan Zhou, the lead author of the Science study, said the efficiency of LANP's copper-ruthenium catalyst derives from a light-induced electronic process that produces localized energy at ruthenium reaction sites, which aids desorption.
The process, known as "hot carrier-driven photocatalysis," has its origins in the sea of electrons that constantly swirl through the copper nanoparticles. Some wavelengths of incoming light resonate with the sea of electrons and set up rhythmic oscillations called localized surface plasmon resonances.
Zhou, a chemist, spent months refining the synthesis of the copper-ruthenium nanoparticles, which are much smaller than a red blood cell. Each nanoparticle contains tens of thousands of copper atoms but just a few thousand ruthenium atoms, which take the place of some copper atoms on the particle's surface.
"Basically, there are ruthenium atoms scattered in a sea of copper atoms, and it's the copper atoms that are absorbing the light, and their electrons are shaking back and forth collectively," Swearer said. "Once a few of those electrons gain enough energy through a quantum process called nonradiative plasmon decay, they can localize themselves within the ruthenium sites and enhance catalytic reactions.
"Room temperature is about 300 Kelvin and plasmon resonances can raise the energy of these hot electrons up to 10,000 Kelvin, so when they localize on the ruthenium, that energy can be used to break the bonds in molecules, assist in adsorption and more importantly in desorption," Swearer said.
Just as a metal picnic table heats up on a sunny afternoon, the white laser light -- a stand-in for sunlight in Zhou's experiments -- also caused the copper-ruthenium catalyst to heat. Because there is no way to directly measure how many hot carriers were created in the particles, Zhou used a heat-sensing camera and spent months taking painstaking measurements to tease apart the thermal-induced catalytic effects from those induced by hot carriers.
"About 20 percent of the light energy was captured for ammonia decomposition," Zhou said. "This is good, and we think we can refine to improve this and make more efficient catalysts."
Zhou and Halas said the team is already working on follow-up experiments to see if other catalytic metals can be substituted for ruthenium, and the initial results are promising.
"Now that we have insight about the specific role of hot carriers in plasmon-mediated photochemistry, it sets the stage for designing energy-efficient plasmonic photocatalysts for specific applications," Halas said.
Original publication
Zhou, Linan and Swearer, Dayne F. and Zhang, Chao and Robatjazi, Hossein and Zhao, Hangqi and Henderson, Luke and Dong, Liangliang and Christopher, Phillip and Carter, Emily A. and Nordlander, Peter and Halas, Naomi J.; "Quantifying hot carrier and thermal contributions in plasmonic photocatalysis"; Science; 2018
Other news from the department science
These products might interest you
Most read news
More news from our other portals
See the theme worlds for related content
Topic world Synthesis
Chemical synthesis is at the heart of modern chemistry and enables the targeted production of molecules with specific properties. By combining starting materials in defined reaction conditions, chemists can create a wide range of compounds, from simple molecules to complex active ingredients.
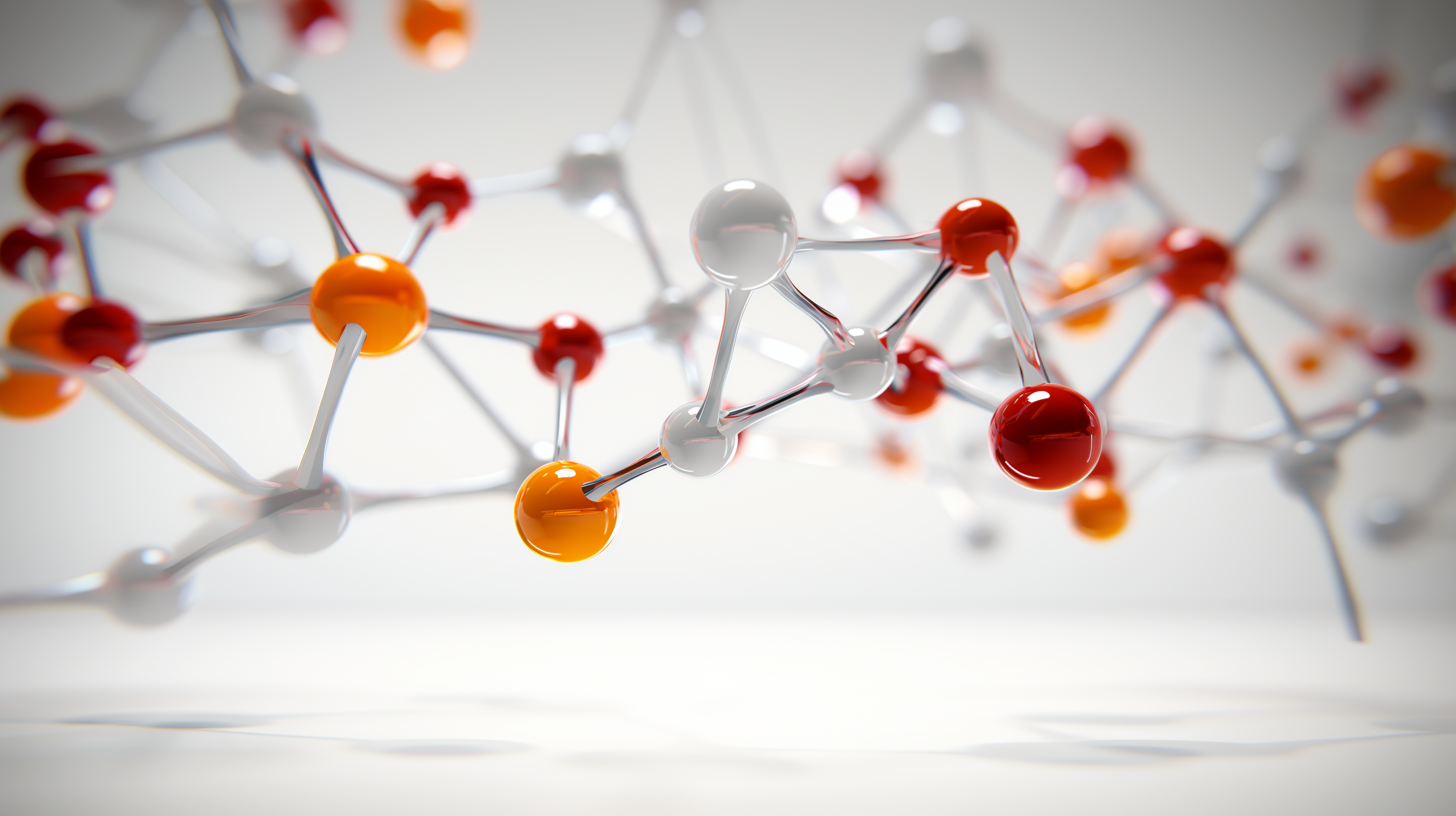
Topic world Synthesis
Chemical synthesis is at the heart of modern chemistry and enables the targeted production of molecules with specific properties. By combining starting materials in defined reaction conditions, chemists can create a wide range of compounds, from simple molecules to complex active ingredients.