How water hides its quantum secret
Researchers show experimentally why water and heavy water behave similarl
Almost three-quarters of the earth are covered by water. Water is the most abundant, but also strangest liquid - for example, it has its maximum density at 4 °C. Researchers around Yuki Nagata and Mischa Bonn at the MPI for Polymer Research have now studied normal water - H2O - and heavy water - D2O - in the laboratory and found out why the two elements behave similarly, even though deuterium atoms (D) are twice as heavy as hydrogen atoms (H). Their investigations can explain, for example, why the freezing points of the two types of water are closer together than one would initially expect.
More than 70 percent of the earth is covered with water - H2O. An almost negligible proportion of a few per mill consists of “heavy water”, in which the hydrogen atoms (H) are replaced by deuterium atoms (D). Deuterium has one more neutron in its nucleus than hydrogen and weighs about twice as much.
One would therefore expect that heavy water, in which two atoms that are twice as heavy are bound to the oxygen atom, would differ significantly in its properties from normal water. In fact, however, the freezing temperatures of the two types of water differ by only 4 °C. Researchers at the Max Planck Institute for Polymer Research around Director Mischa Bonn have now been able to show for the first time that two special quantum mechanical effects – so-called nuclear quantum effects (NQE) – which compensate each other, are responsible for this.
Quantum mechanics dictates that atoms continue to move even at absolute zero, i.e. at -273 °C: They "oscillate" or "tremble" slightly around a central position. This is referred to as "zero-point energy". The hydrogen atoms in normal water are, therefore, not at a defined, fixed distance from the oxygen atom but in a certain "cloud," which extends around an average distance. Because hydrogen is such a low-mass atom, these hydrogen clouds are large, NQEs are pronounced, and the vibration has a large amplitude.
If hydrogen is replaced by the heavier deuterium, the atoms vibrate less. The mean distance becomes smaller, meaning that the deuterium atom moves closer to the oxygen atom. As a result of this so-called intramolecular effect, the spatial expansion of a water molecule is reduced. Simultaneously, the distance to the next water molecule increases, which reduces the binding energy.
The binding energy is a measure of how easily two water molecules can be separated from each other - for example, during the transition from ice to liquid water.
At the same time, however, the deuterium atom can oscillate not only in the direction of the bond line to the oxygen atom, but also perpendicular to it. When exchanging hydrogen for deuterium, this so-called intermolecular effect counteracts the intramolecular effect: while one reduces the binding energy, the other increases the binding energy to a comparable extent.
The freezing temperatures differ only slightly because the two quantum mechanical effects have opposite effects on the binding energy and approximately compensate each other.
To measure these subtle effects, the researchers used a technique called heterodyne-detected sum-frequency generation (HD-SFG) spectroscopy. This method allowed them to study the uppermost water layer at an air-water interface, where water molecules exist with a “free” end that is not attached to other water molecules. By carefully analyzing the vibrational spectra of water with different proportions of hydrogen and deuterium, the scientists were able to deduce and quantify the individual inter- and intramolecular energy components.
The work, now published in the journal Science Advances, provides the first experimental evidence of the competition, and almost complete cancellation between intramolecular and intermolecular quantum effects in water, which has long been predicted only theoretically. It highlights the importance of considering these quantum phenomena when trying to understand the behavior of water. This has implications for fields ranging from climate research to biochemistry, where the properties of water play a crucial role. Furthermore, the team's innovative approach opens up new avenues for studying quantum effects in other complex systems.
Original publication
Other news from the department science
Most read news
More news from our other portals
See the theme worlds for related content
Topic World Spectroscopy
Investigation with spectroscopy gives us unique insights into the composition and structure of materials. From UV-Vis spectroscopy to infrared and Raman spectroscopy to fluorescence and atomic absorption spectroscopy, spectroscopy offers us a wide range of analytical techniques to precisely characterize substances. Immerse yourself in the fascinating world of spectroscopy!
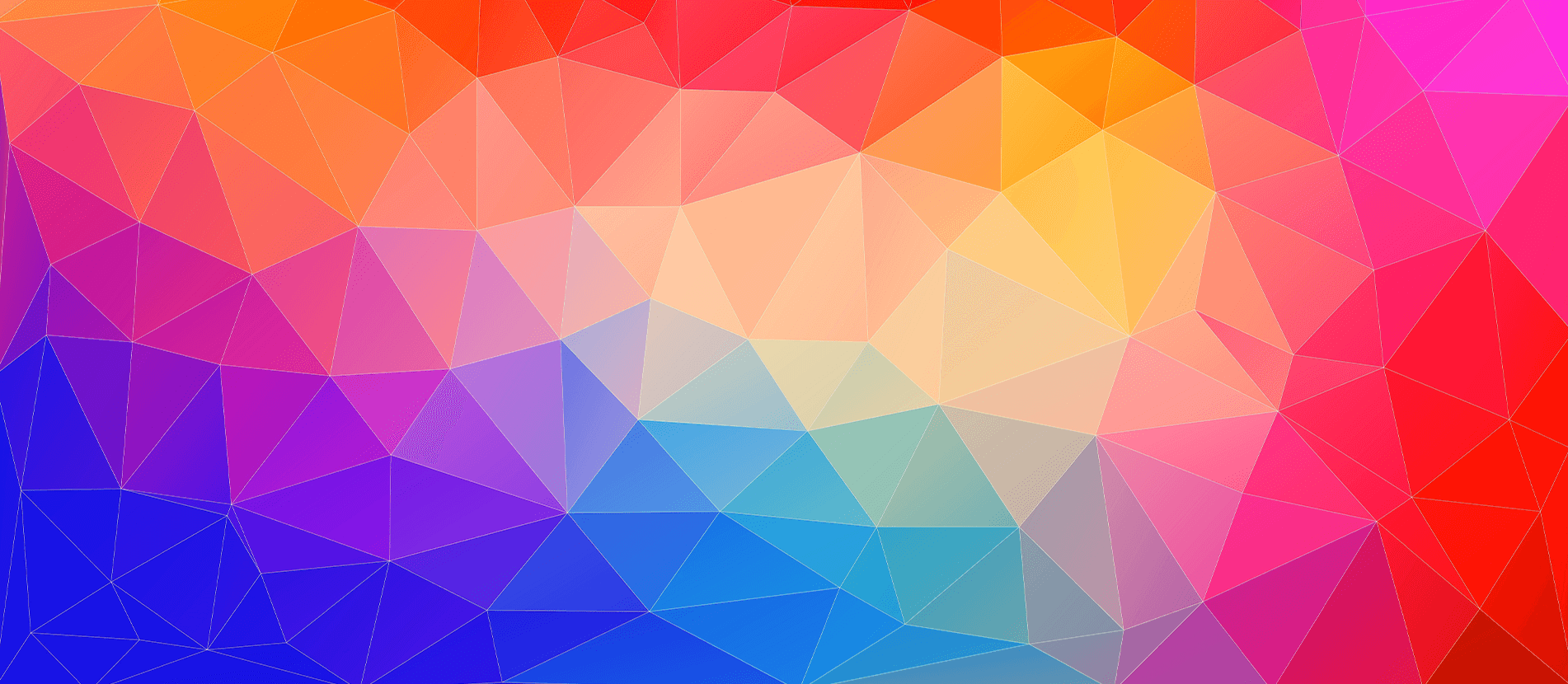
Topic World Spectroscopy
Investigation with spectroscopy gives us unique insights into the composition and structure of materials. From UV-Vis spectroscopy to infrared and Raman spectroscopy to fluorescence and atomic absorption spectroscopy, spectroscopy offers us a wide range of analytical techniques to precisely characterize substances. Immerse yourself in the fascinating world of spectroscopy!