AI tackles one of the most difficult challenges in quantum chemistry
New research using neural networks, a form of brain-inspired AI, proposes a solution to the tough challenge of modelling the states of molecules. The research shows how the technique can help solve fundamental equations in complex molecular systems. This could lead to practical uses in the future, helping researchers to prototype new materials and chemical syntheses using computer simulation before trying to make them in the lab.Led by Imperial College London and Google DeepMind scientists, the study is published in Science.
Excited molecules
The team investigated the problem of understanding how molecules transition to and from ‘excited states’. When molecules and materials are stimulated by a large amount of energy, such as being exposed to light or high temperatures, their electrons can get kicked into a temporary new configuration, known as an excited state.
The exact amount of energy absorbed and released as molecules transition between states creates a unique fingerprint for different molecules and materials. This affects the performance of technologies ranging from solar panels and LEDs to semiconductors and photocatalysts. They also play a critical role in biological processes involving light, including photosynthesis and vision.
However, this fingerprint is extremely difficult to model because the excited electrons are quantum in nature, meaning their positions within the molecules are never certain, and can only be expressed as probabilities.
Lead researcher Dr David Pfau, from Google DeepMind and the Department of Physics at Imperial, said: “Representing the state of a quantum system is extremely challenging. A probability has to be assigned to every possible configuration of electron positions.
“The space of all possible configurations is enormous — if you tried to represent it as a grid with 100 points along each dimension, then the number of possible electron configurations for the silicon atom would be larger than the number of atoms in the universe. This is exactly where we thought deep neural networks could help.”
Neural networks
The researchers developed a new mathematical approach and used it with a neural network called FermiNet (Fermionic Neural Network), which was the first example where deep learning was used to compute the energy of atoms and molecules from fundamental principles that was accurate enough to be useful.
The team tested their approach with a range of examples, with promising results. On a small but complex molecule called the carbon dimer, they achieved a mean absolute error (MAE) of 4 meV (millielectronvolt – a tiny measure of energy), which is five times closer to experimental results than prior gold standard methods reaching 20 meV.
Dr Pfau said: “We tested our method on some of the most challenging systems in computational chemistry, where two electrons are excited simultaneously, and found we were within around 0.1 eV of the most demanding, complex calculations done to date.
“Today, we’re making our latest work open source, and hope the research community will build upon our methods to explore the unexpected ways matter interacts with light.”
Original publication
Other news from the department science
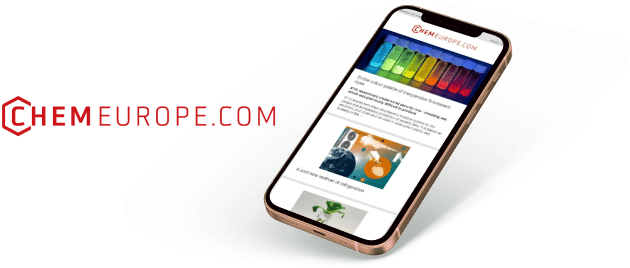
Get the chemical industry in your inbox
From now on, don't miss a thing: Our newsletter for the chemical industry, analytics, lab technology and process engineering brings you up to date every Tuesday and Thursday. The latest industry news, product highlights and innovations - compact and easy to understand in your inbox. Researched by us so you don't have to.