Room Temperature Conversion of CO₂ to CO: A New Way to Synthesize Hydrocarbons
New method could potentially reduce dioxide emission into the atmosphere and slash costs of chemical manufacturing
Researchers at the National Institute of Standards and Technology (NIST) and their colleagues have demonstrated a room-temperature method that could significantly reduce carbon dioxide levels in fossil-fuel power plant exhaust, one of the main sources of carbon emissions in the atmosphere.
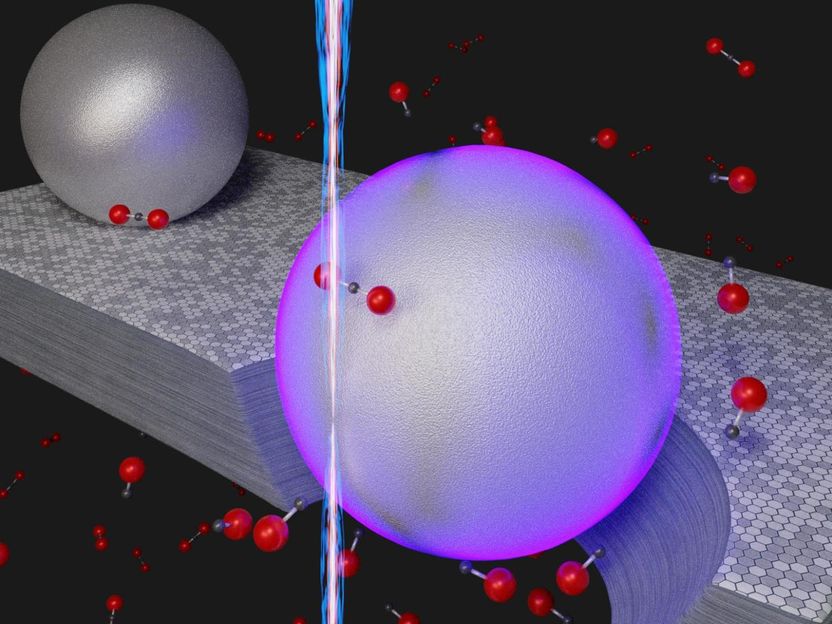
Illustration of a novel room-temperature process to remove carbon dioxide (CO₂) by converting the molecule into carbon monoxide (CO). Instead of using heat, the nanoscale method relies on the energy from surface plasmons (violet hue) that are excited when a beam of electrons (vertical beam) strikes aluminum nanoparticles resting on graphite, a crystalline form of carbon. In the presence of the graphite, aided by the energy derived from the plasmons, carbon dioxide molecules (black dot bonded to two red dots) are converted to carbon monoxide (black dot bonded to one red dot. The hole under the violet sphere represents the graphite etched away during the chemical reaction CO₂ + C = 2CO.
NIST
Although the researchers demonstrated this method in a small-scale, highly controlled environment with dimensions of just nanometers (billionths of a meter), they have already come up with concepts for scaling up the method and making it practical for real-world applications.
In addition to offering a potential new way of mitigating the effects of climate change, the chemical process employed by the scientists also could reduce costs and energy requirements for producing liquid hydrocarbons and other chemicals used by industry. That's because the method's byproducts include the building blocks for synthesizing methane, ethanol and other carbon-based compounds used in industrial processing.
The team tapped a novel energy source from the nanoworld to trigger a run-of-the-mill chemical reaction that eliminates carbon dioxide. In this reaction, solid carbon latches onto one of the oxygen atoms in carbon dioxide gas, reducing it to carbon monoxide. The conversion normally requires significant amounts of energy in the form of high heat -- a temperature of at least 700 degrees Celsius, hot enough to melt aluminum at normal atmospheric pressure.
Instead of heat, the team relied on the energy harvested from traveling waves of electrons, known as localized surface plasmons (LSPs), which surf on individual aluminum nanoparticles. The team triggered the LSP oscillations by exciting the nanoparticles with an electron beam that had an adjustable diameter. A narrow beam, about a nanometer in diameter, bombarded individual aluminum nanoparticles while a beam about a thousand times wider generated LSPs among a large set of the nanoparticles.
In the team's experiment, the aluminum nanoparticles were deposited on a layer of graphite, a form of carbon. This allowed the nanoparticles to transfer the LSP energy to the graphite. In the presence of carbon dioxide gas, which the team injected into the system, the graphite served the role of plucking individual oxygen atoms from carbon dioxide, reducing it to carbon monoxide. The aluminum nanoparticles were kept at room temperature. In this way, the team accomplished a major feat: getting rid of the carbon dioxide without the need for a source of high heat.
Previous methods of removing carbon dioxide have had limited success because the techniques have required high temperature or pressure, employed costly precious metals, or had poor efficiency. In contrast, the LSP method not only saves energy but uses aluminum, a cheap and abundant metal.
Although the LSP reaction generates a poisonous gas -- carbon monoxide -- the gas readily combines with hydrogen to produce essential hydrocarbon compounds, such as methane and ethanol, that are often used in industry, said NIST researcher Renu Sharma.
She and her colleagues, including scientists from the University of Maryland in College Park and DENSsolutions, in Delft, the Netherlands, reported their findings in Nature Materials.
"We showed for the first time that this carbon dioxide reaction, which otherwise will only happen at 700 degrees C or higher, can be triggered using LSPs at room temperature," said researcher Canhui Wang of NIST and the University of Maryland.
The researchers chose an electron beam to excite the LSPs because the beam can also be used to image structures in the system as small as a few billionths of a meter. This enabled the team to estimate how much carbon dioxide had been removed. They studied the system using a transmission electron microscope (TEM).
Because both the concentration of carbon dioxide and the reaction volume of the experiment were so small, the team had to take special steps to directly measure the amount of carbon monoxide generated. They did so by coupling a specially modified gas cell holder from the TEM to a gas chromatograph mass spectrometer, allowing the team to measure parts-per-millions concentrations of carbon dioxide.
Sharma and her colleagues also used the images produced by the electron beam to measure the amount of graphite that was etched away during the experiment, a proxy for how much carbon dioxide had been taken away. They found that the ratio of carbon monoxide to carbon dioxide measured at the outlet of the gas cell holder increased linearly with the amount of carbon removed by etching.
Imaging with the electron beam also confirmed that most of the carbon etching -- a proxy for carbon dioxide reduction -- occurred near the aluminum nanoparticles. Additional studies revealed that when the aluminum nanoparticles were absent from the experiment, only about one-seventh as much carbon was etched.
Limited by the size of the electron beam, the team's experimental system was small, only about 15 to 20 nanometers across (the size of a small virus).
To scale up the system so that it could remove carbon dioxide from the exhaust of a commercial power plant, a light beam may be a better choice than an electron beam to excite the LSPs, Wang said. Sharma proposes that a transparent enclosure containing loosely packed carbon and aluminum nanoparticles could be placed over the smokestack of a power plant. An array of light beams impinging upon the grid would activate the LSPs. When the exhaust passes through the device, the light-activated LSPs in the nanoparticles would provide the energy to remove carbon dioxide.
The aluminum nanoparticles, which are commercially available, should be evenly distributed to maximize contact with the carbon source and the incoming carbon dioxide, the team noted.
The new work also suggests that LSPs offer a way for a slew of other chemical reactions that now require a large infusion of energy to proceed at ordinary temperatures and pressures using plasmonic nanoparticles.
"Carbon dioxide reduction is a big deal, but it would be an even bigger deal, saving enormous amounts of energy, if we can start to do many chemical reactions at room temperature that now require heating," Sharma said.