Researchers couple artificial atom to acoustic resonator
Researchers from Russia and Britain have demonstrated an artificial quantum system, in which a quantum bit interacts with an acoustic resonator in the quantum regime. This allows the familiar effects of quantum optics to be studied on acoustic waves and enables an alternative approach to quantum computer design, which is based on acoustics and could make quantum computers more stable and compact.
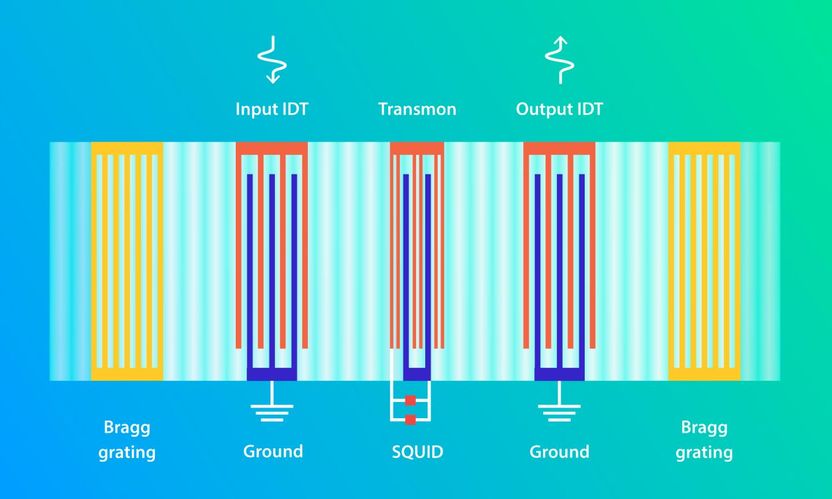
Schematic of the chip. The resonator is a Fabry-Perot cavity formed by two Bragg gratings, each consisting of 200 parallel stripes (shown in yellow) separated by half the acoustic wavelength. The wavelength is equal to 0.98 micrometers, or 980 nanometers. There are two interdigital transducer (IDT) ports -- a receiver and a transmitter -- and a qubit (transmon) inside the resonator. SQUID is the part of the transmon sensitive to weak magnetic fields.
Elena Khavina/MIPT Press office and the researchers
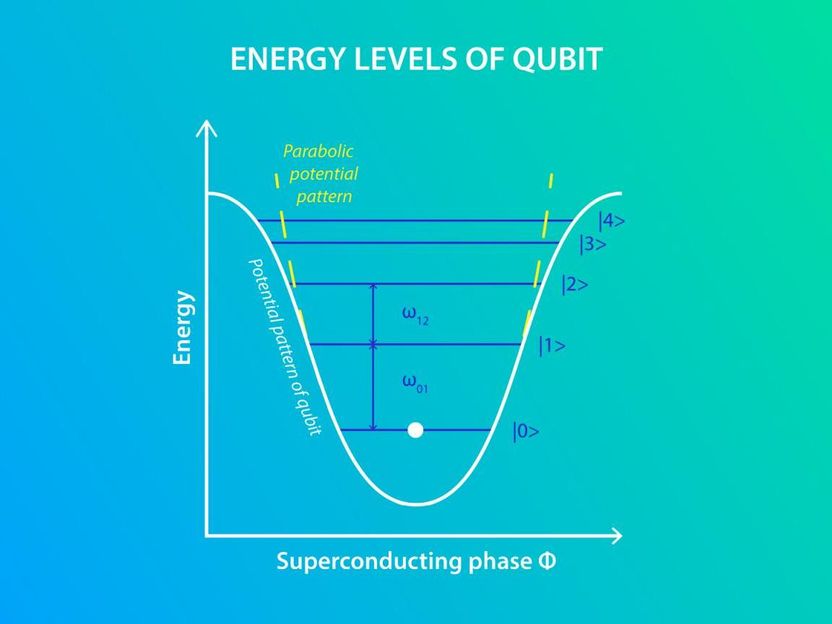
The energy spectrum of a transmon is similar to that of an atom. The frequency of the transition between the first two energy levels is ω01.
Elena Khavina/MIPT Press office and the researchers
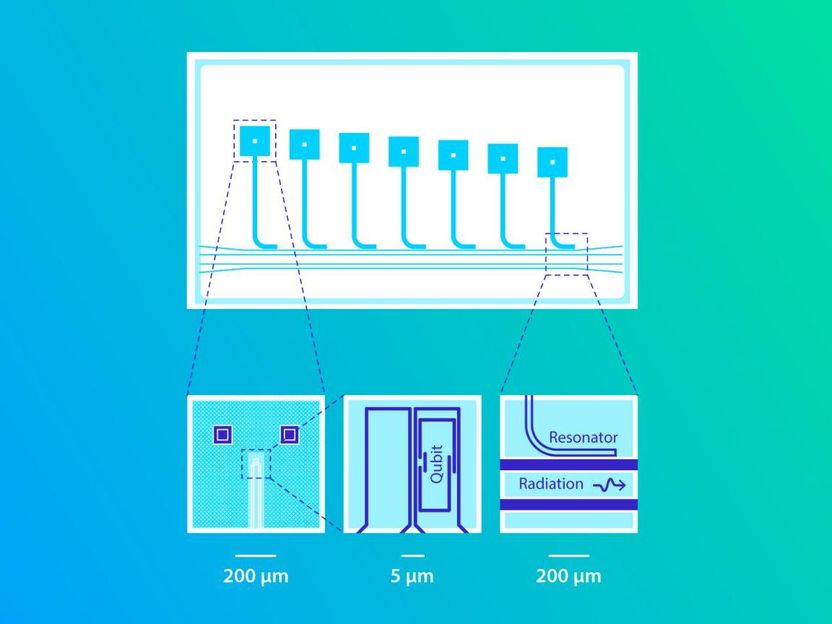
Microwave chip. Each of the seven square regions in the top picture contains a qubit. The L-shaped lines in the top picture are microwave resonators, each of which has a distinct resonant frequency. A micrometer (1 μm) is one-millionth of a meter.
Elena Khavina/MIPT Press office and the researchers
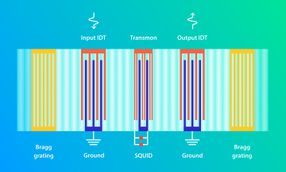
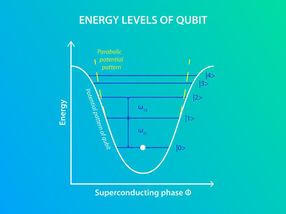
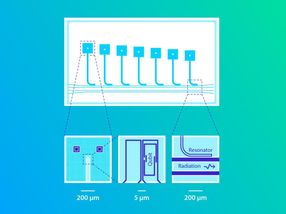
"We are the first to demonstrate an interaction between a qubit and a surface acoustic wave resonator in the quantum regime. Previously, resonators of this kind were studied, but without a qubit. Likewise, qubits with surface acoustic waves were studied, but those were running waves, without a resonator. The quantum regime was demonstrated on bulk resonators, but this didn't go far, perhaps due to difficulties in fabrication. We used a planar structure fabricated with existing technologies," says Aleksey Bolgar, researcher at MIPT's Artificial Quantum Systems Lab, where the study was conducted.
The researchers studied the interaction of a superconducting qubit, a transmon, with surface acoustic waves in a resonator (figure 1). The transmon behaves as an artificial atom -- that is, it has a number of energy levels (figure 2) and undergoes transitions between them. The conventional microwave approach is to have one chip holding both the qubit and a microwave resonator supporting and amplifying the wave. In this setup, the qubit can interact with the resonator either by absorbing a photon from it and entering an excited state or by emitting a photon into it and returning to the ground state, provided that the photon frequency corresponds to the transition frequency of the qubit. The resonant frequency of the resonator itself varies depending on the state of the qubit. Therefore, by changing resonator characteristics, it is possible to read information from the qubit.
An alternative approach has recently emerged. Instead microwave radiation (photons), it uses mechanical excitations, or phonons, in the form of acoustic waves. This quantum acoustic approach has been developed to a much lesser extent, compared with its microwave counterpart, but it has a number of advantages.
Since acoustic waves propagate 100,000 times slower than light, their wavelength is accordingly shorter. The size of a resonator needs to "fit" the wavelength used. In a microwave quantum system, the wavelength is about 1 centimeter at best. This means that the resonator needs to be fairly large, but the larger it is, the more defects it has, since they are inevitably present on the surface of the chip. Due to these defects, the lifetime of a qubit state is short, impairing large-scale quantum calculations and complicating the creation of quantum computers. As of now, the world record for the longest lifetime is around 100 microseconds, or one-ten-thousandth of a second. Under the acoustic approach, the wavelength equals roughly 1 micrometer, so it is possible to fit high-quality resonators measuring just 300 micrometers on the chip.
Another problem with microwaves is that the long wavelengths make it impossible to put two qubits into one resonator to enable interaction at different frequencies. As a result, a separate resonator is needed for each qubit (see figure 3). In the acoustic approach, one mechanical resonator can accommodate several qubits with slightly different transition frequencies. This means a quantum chip based on sound waves would be much smaller than those available now. Moreover, acoustodynamics could resolve the issue of quantum system sensitivity to electromagnetic noise.
The authors of the paper used a resonator for surface acoustic waves. These are somewhat similar to seawaves but propagating in solids. Figure 4 shows the chip created in the study. An aluminum circuit is deposited on a piezoelectric substrate made of quartz. The circuit consists of a transmon, a resonator, and two interdigital transducers. The two transducers serve as a transmitter and a receiver. Between them, there is a piezoelectric layer made of a material that converts mechanical stress into electricity and vice versa. A surface acoustic wave generated on the piezoelectric material is caught between the two Bragg gratings of the resonator. The qubit, or transmon, contained in the resonator has two energy levels, and the qubit capacitance is implemented as interdigital transducers. The purpose of the study was to show that the qubit can interact with the resonator, becoming excited and relaxed the way a quantum system would. The measurements were made in a cryostat under temperatures in the tens of millikelvins.
A characteristic feature of the quantum regime is the so-called avoided crossing of energy levels (figure 5). The transition frequency of the qubit can be tuned via an external magnetic field -- to enable this, the transmon is equipped with a SQUID magnetometer. If the frequency of the resonator coincides with the qubit transition frequency, energy splitting is observed in the energy spectrum of the qubit -- that is, one magnetic flux value corresponds to two characteristic transition frequencies. The researchers observed this phenomenon in their chip and showed that the transmon and the acoustic resonator interact in the quantum regime.
The fundamental goal of this research is to demonstrate that the phenomena and effects of quantum optics apply to acoustics as well. In addition, it provides an alternative way to build a quantum computer. Despite microwave-based interfaces achieving an impressive 50-qubit count, which means quantum acoustics still has a long way to go, the latter approach has numerous advantages that might come in handy in the future.
Apart from the staff of MIPT's Artificial Quantum Systems Lab, researchers from MISIS National University for Science and Technology, Moscow State Pedagogical University, and Royal Holloway, University of London, participated in the study.
This research was carried out using the technological equipment of MIPT and was supported by the Russian Science Foundation and the Ministry of Education and Science of Russia.