Ultracold atoms in a "Rydberg-dress"
Scientists have developed a novel technique to let atoms interact over large distances
Many properties of our everyday world can be explained if atoms are thought of as small, solid marbles, which feel each other only if brought in direct contact with each other. The temperature of the air surrounding us, for example, is the result of uncountable, continuously occurring collisions between its constituents. Contrary to this, we also know effects which arise from the interplay between two distant objects. Well-known examples are two magnets which can affect each other also at quite a distance, or the formation of a salt crystal as a regular arrangement of positively charged sodium and negatively charged chlorine ions, which are bound together at large distances by electrical attraction.
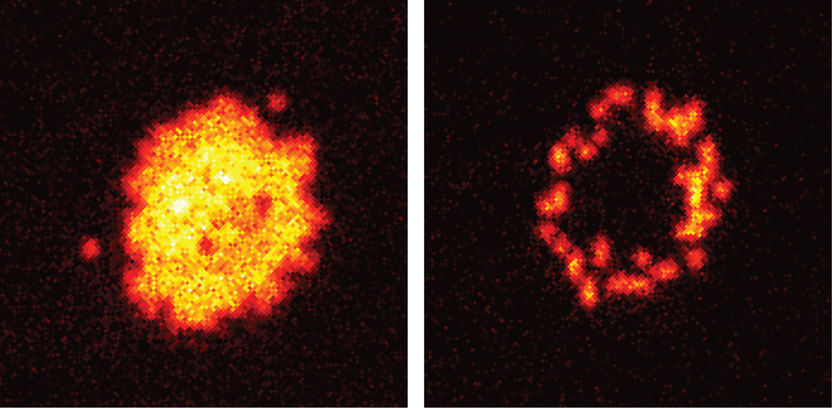
Fig. 1: From the starting state densely filled with atoms (left), a ring-like structure emerges due to the long range interaction (right).
MPQ, Quantum Many-Body Systems Division
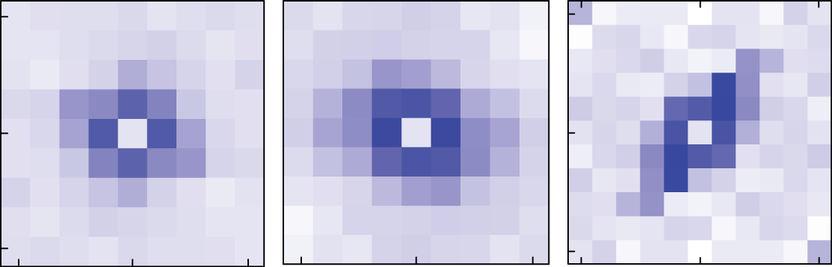
Fig. 2: The type of interaction can be controlled by light, from angularly independent (left and middle) to angularly dependent interaction (right).
MPQ, Quantum Many-Body Systems Division
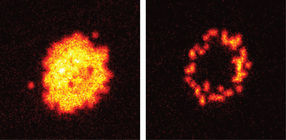
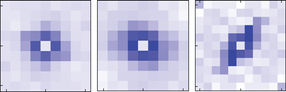
In the microscopic quantum world, such interactions at a distance are of special interest, as on the one hand they are the origin of foundational, well known phenomena such as the formation of ordered crystals. On the other hand they also promise to allow for experimentally studying novel and up to now unknown states of matter. Moreover, such long-range interacting systems are hard to treat theoretically on a fundamental level, attaching special value to experimental studies.
Now, a team of researchers around Dr. Christian Groß and Prof. Immanuel Bloch (MPQ Garching) in collaboration with Dr. Thomas Pohl (MPIPKS Dresden) has developed a novel method to let atoms interact over a large distance. The key element thereby is the so called “Rydberg-dressing”, which makes use of a fundamental property of quantum mechanics, namely the fact that a quantum object can be in a superposition of two states at the same time. To illustrate this phenomenon, one often quotes the famous “Schrödinger’s cat”, devised by the theoretical physicist Erwin Schrödinger, which is held in a closed box in a superposition of the states “dead” and “alive”. In analogy, in the experiment atoms are brought into a superposition of two states. “The trick consists of choosing a highly excited “Rydberg-state” next to the energetically lowest lying ground state”, explains Johannes Zeiher, doctoral candidate at the experiment. “These exotic states are characterized by a 1000-fold increased diameter. Therefore, Rydberg atoms can influence each other at enormous distances.” The catch, however, is that Rydberg-atoms are unstable and decay within a very short time. The scientists take this hurdle by choosing a very unequal superposition, in which the atoms are in the unstable Rydberg state only with a small probability. “To some extent, each atom only obtains a very thin “Rydberg-dress”, which nevertheless can be experienced by other, distant atoms”, elucidates Christian Groß, leader of the experiment.
In the laboratory, the physicists start the experiment by creating an ultracold gas of the alkali metal rubidium-87 with the aid of the technique of laser-cooling. From this gas, approximately 200 atoms are transferred to a so called optical lattice, a periodic arrangement of microscopic light traps, which are formed by several laser beams. Within a single plane, each of these microscopic traps is dimensioned such that it can hold precisely one atom. The resulting order of the atoms is a well-controlled starting state for the next, decisive step: the implementation of “Rydberg-dressing” by shining in very intense, ultraviolet laser light. In this light-woven “Rydberg-dress”, the atoms started feeling and affecting each other at a distance, similar to two magnets repelling or attracting each other at a much larger scale in our macroscopic world. A crucial difference is, however, that in the microscopic system this interaction can be switched on and off by controlling the ultraviolet light.
To provide the proof for the so generated long range interactions, the experimentalist chose an interferometric technique which allows for an especially sensitive probe of the system. To this end, the “dressed atoms”, for which the ground state is superposed with the Rydberg state, are compared to usual atoms. The mutual attraction or repulsion of the Rydberg atoms leaves characteristic footprints in the interference pattern arising from this comparison. These can be detected by imaging the atoms one by one with the aid of a specialized fluorescence microscope.
A first measurement provided direct evidence that the atoms feel each other at large distances. As a consequence, the behaviour of each atom is also influenced by its neighbours. Figure 1 shows both the initial distribution of about 200 atoms, which homogeneously occupy a disc shaped area, as well as the resulting interference pattern for the atoms in superposition with the Rydberg state. The edge of the system stands out as a ring-like structure, as the atoms there lack their neighbours at the outside.
A deeper analysis of the structures in the interference pattern allowed for a more precise measurement and characterization of the interaction. The experiments confirm with excellent accuracy the theoretical predictions. A particularly interesting feature is the possibility to create angularly dependent interactions (Figure 2). This means that two atoms lying next to each other feel each other differently depending on whether they follow each other from left to right or orthogonal to this direction.
“This phenomenon also can be observed with two magnets, which repel or attract each other with different strength, depending on whether they are arranged next to each other or before each other”, says Christian Groß. Contrary to this, the interaction underlying the crystal formation of sodium and chlorine ions is independent of the angle. Also this more simple type of interaction could be realized in the lab, controlled by the ultraviolet laser (Figure 2).
The research teams around Immanuel Bloch, Christian Groß and Thomas Pohl succeeded in inducing and characterizing a novel form of interaction between two atoms. Control over this interaction with the aid of light opens the path to study microscopic systems, in which atoms act like small magnets and interact over large distances. Such systems promise studies of a large variety of exciting phenomena, for example of the thus far not experimentally observed “Super-solid”, which is a state of matter that is a solid and a fluid at the same time.