Nanoelectronics researchers employ Titan for an electrifying simulation speedup
The team, led by Mathieu Luisier from ETH Zurich, focuses on further developing the front line of electronics research - simulating and better understanding nanoscale components such as transistors or battery electrodes whose active regions can be on the order of one-billionth of a meter, or about as long as your fingernails grow in one second.
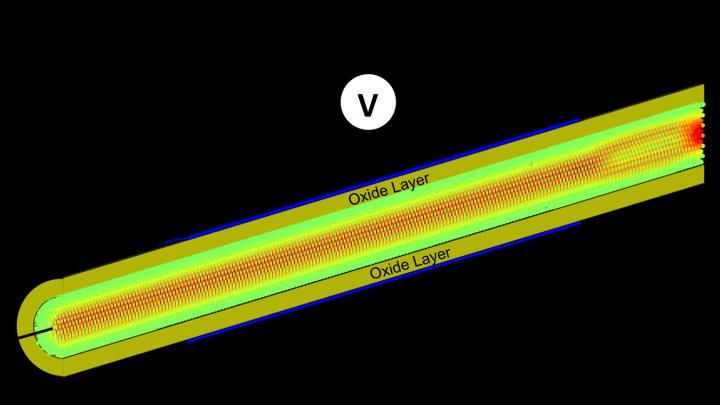
Spatial distribution of the electron current flowing through a silicon gate-all-around nanowire field-effect transistor composed of 55,488 atoms. A voltage (V) is applied to the structure. Half of the oxide-capping layer is removed to shed light on the interior of the transistor where the atoms are colored according to the current they carry: green means no current, whereas red indicates a high concentration.
Mathieu Luisier, ETH Zurich
Though the scales of the investigated objects are small, the team has made big progress toward more efficient computational codes. Its research was selected as a finalist for this year's Association of Computing Machinery's Gordon Bell Prize, one of the most prestigious awards in supercomputing.
"Our goal is to study nanoscale devices, such as nanotransistors, batteries or a variety of other new devices such as computer memories, optical switches or light emitting diodes on an atomic level," Luisier said. "If you want to make these simulations accurate and truly predictive, you need to use so-called ab initio, or from first principles, simulation methods."
Researchers studying nanoelectronics thus typically have to make a compromise between simulating a realistic system size (at least 10,000 atoms) and using highly accurate ab initio methods.
To this point, though, most ab initio software packages focus on the calculation of material properties such as crystal and electronic structures, lattice vibrations, or phase diagrams and do not account for the real operating conditions - under the application of external voltage, an electron current starts to flow through active nanostructures. These transport phenomena are computationally very demanding and require a dedicated modeling approach.
Luisier and his team, therefore, developed a method for doing ab initio transport simulations that are large enough to investigate nanostructures with sizes relevant to industry and experimental groups. They just needed the right machine to test it.
Two partner codes, one objective
Luisier explained that there are two main challenges for simulating transport through nanoelectronic components. First, researchers must calculate what they call open boundary conditions that couple the simulation with its surrounding environment and enable current flows. As a second step, they must incorporate the created boundary blocks into the Hamiltonian, a matrix that contains all of the interatomic interactions characterizing the device, and finally they must solve the resulting sparse linear system of equations. Using this approach, typical state-of-the-art simulations within the field can accurately model around 1,000 atoms.
"What allowed us to get so much faster and treat really large device structures is that we found a way to efficiently perform most of the work, solving the linear system, on Titan's compute nodes, using extremely fast GPUs, while still keeping the CPUs busy with computing the boundary conditions at the same time," Luisier said.
The team first tested its method on the Swiss National Supercomputing Centre's Piz Daint machine, growing the simulation from 1,000 atoms to 15,000. For Luisier, this was extremely encouraging, but he believed the team could do more.
After these initial and successful runs, the team received time on Titan as part of the Director's Discretionary program. Moving from Piz Daint, with its 5,000-plus compute nodes, to Titan - with upwards of 18,000 nodes - allowed the team to perform a simulation with 50,000 atoms, easily beating the prior benchmark. Luisier also noted that getting to a 50,000-atom simulation did not even use all of Titan's supercomputing power, meaning that larger simulations are not just theoretical, but likely, in the near future.
By finding a method to do ab initio quantum transport calculations on such a large system, the team is the first to run simulations that can correspond with experiments in the field, potentially helping advance research and development for next-generation electronic devices.
"If you just have 1,000 atoms, you can't really simulate a real device," Luisier said. "That would require simulating about 10 times as many of them. With the new method, we can really model something that looks like a transistor or a storage unit at the ab initio level. And the nanowires that we've been investigating have already been fabricated around 10 years ago when experimentalists were not as advanced in producing small structures as they are now. So the maximum of what we can now simulate goes beyond the smallest structures people can actually manufacture in the lab today."
Though the codes' sustained performance is impressive -15 petaflops, or 15 quadrillion calculations per second - Luisier emphasized that these simulations were not performed to set new computational performance benchmarks in the field, but rather were to further research.
"This is really a production code, a code that is used on a day-to-day basis," Luisier said. "What comes out of these runs is not just FLOPS on a computer - these results are used in collaboration with experimentalists at ETH Zürich and abroad. There are a couple of groups very interested in the results because they can explain what these groups observe in their experimental devices -not only in nanotransistors but also in light-emitting components or quantum dot solar cells, to cite just a few examples."