Liquids and glasses relax, too. But not like you thought.
A new insight into the fundamental mechanics of the movement of molecules published by researchers at the National Institute of Standards and Technology (NIST) offers a surprising view of what happens when you pour a liquid out of a cup. More important, it provides a theoretical foundation for a molecular-level process that must be controlled to ensure the stability of important protein-based drugs at room temperature.
Proteins depend critically on their three-dimensional structure, the shape the long and complex molecules tend to fold into. Modern protein-based drugs - or example, vaccines or antibodies created to fight cancers - generally are not stable at room temperature or in the liquid formulations most convenient for clinical use. To preserve them for use in parts of the world without reliable refrigeration, manufacturers freeze-dry the proteins and coat the complex molecules with glassy sugars to keep their structure intact. "It's like a lollypop," observes NIST biochemist Marcus Cicerone, "but these lollypops are only 10 microns or smaller."
The challenge is to design the sugar coating to get the maximum shelf life for a given pharmaceutical protein, which ideally would be measured in years. The issue revolves around what chemists refer to as "relaxation"--broadly, any molecular motion that leads to transport of the molecule. About 10 years ago, NIST researchers discovered a testing shortcut. Using neutron radiation, they discovered that measuring tiny molecular movements in the proteins at very short timescales - picoseconds - could reliably predict the long-term stability of a formulation. The sugars that worked the best were the ones that suppressed the tiny, rapid motions. Exactly why this was so was not particularly clear, but it worked.
This new paper finally explains the underlying principles. The neutron experiments, says Cicerone, measure mean square displacement. "Imagine a jarful of molecules. It's how far the average molecule jiggles around for a given timescale," he says. "In condensed matter like a liquid or glass, we usually think that all the molecules are identical, and on the average they all have the same environment with a little bit of space for them to jiggle, but not very much."
"What we found is that picture is not really right."
In reality, Cicerone says, there are two different environments the molecules can be in. "There is one environment like that - molecules are very well packed and on a picosecond timescale they move maybe one percent of their radius. They're hardly moving at all. But there's another environment where some molecules can move maybe 30 percent of their radius in the same time.They're really making big jumps, and in glasses, those big jumps are essentially the only way that molecules can move around. Everybody else is completely stuck.
"It's kind of like a 15 puzzle. You can only move one at a time."
What happens is a molecule next to a region that's more loosely packed can move there, and does. Then one that was next to it suddenly has room to move, and does, and so on. On a picosecond and nanometer scale of time and space, when you pour a liquid out of a cup, it doesn't really all come out all at once. It's more follow-the-leader.
On a practical level, says Cicerone, the results explain why the short timescale mean displacement measurements can predict the results of molecular degradation measurements that would normally take months. "It gives a really good solid understanding of why these picosecond and nanosecond timescale measurements correlate with degradation processes in glass for the proteins," he says, "so it gives us confidence that the techniques we build that are based on this idea will be robust and people will be able to use them."
As a bonus, he says, the model also explains a somewhat arcane degradation process in glasses called Johari-Goldstein relaxation. "It's the timescale for the switching between the tightly packed and loosely packed regions. It's the vacancy in the game of 15 moving around," says Cicerone.
Original publication
M.T. Cicerone, Q. Zhong and M. Tyagi; "Picosecond dynamic heterogeneity, hopping and Johar-Goldstein relaxation in glass-forming liquids."; Physical Review Letters 113,117801-117801 (2014).
Original publication
M.T. Cicerone, Q. Zhong and M. Tyagi; "Picosecond dynamic heterogeneity, hopping and Johar-Goldstein relaxation in glass-forming liquids."; Physical Review Letters 113,117801-117801 (2014).
Organizations
Other news from the department science
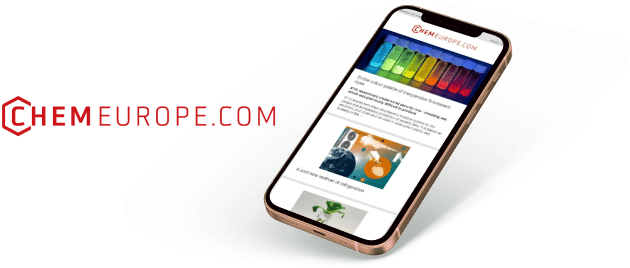
Get the chemical industry in your inbox
By submitting this form you agree that LUMITOS AG will send you the newsletter(s) selected above by email. Your data will not be passed on to third parties. Your data will be stored and processed in accordance with our data protection regulations. LUMITOS may contact you by email for the purpose of advertising or market and opinion surveys. You can revoke your consent at any time without giving reasons to LUMITOS AG, Ernst-Augustin-Str. 2, 12489 Berlin, Germany or by e-mail at revoke@lumitos.com with effect for the future. In addition, each email contains a link to unsubscribe from the corresponding newsletter.