Controlling matter at the atomic level: University of Bath breakthrough
Physicists are getting closer to controlling single-molecule chemical reactions – could this shape the future of pharmaceutical research?
Controlling matter at the atomic level has taken a major step forward, thanks to groundbreaking nanotechnology research by an international team of scientists led by physicists at the University of Bath.
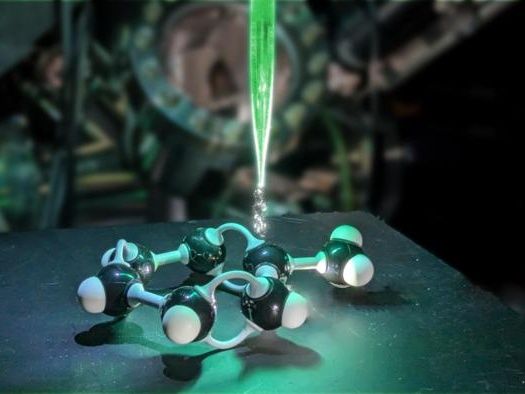
An artist’s representation of a scanning tunnelling microscope probing a toluene molecule
Dr Kristina Rusimova, Hannah Martin and Pieter Keenan
This advancement has profound implications for fundamental scientific understanding. It is also likely to have important practical applications, such as transforming the way researchers develop new medications.
Controlling single-outcome single-molecule reactions is now almost routine in research laboratories across the world. For example, over a decade ago, researchers from the technology giant IBM showcased their ability to manipulate individual atoms by creating A boy and his atom, the world's smallest movie. In the film, single molecules, consisting of two atoms bonded together, were magnified 100-million times and positioned frame-by-frame to tell a stop-motion story on an atomic scale.
Achieving control over chemical reactions with multiple outcomes, however, has remained elusive. This matters because generally only some outcomes of a chemical reaction are useful.
For instance, during drug synthesis, a chemical process that results in ‘cyclisation’ produces the desired therapeutic compound, however ‘polymerisation’, another outcome, leads to unwanted byproducts.
Being able to precisely control reactions to favour desired outcomes and reduce unwanted byproducts promises to improve the efficiency and sustainability of pharmaceutical processes.
Scanning tunnelling microscopy
The new study, published in the journal Nature Communications, set out to demonstrate for the first time that competing chemical reaction outcomes can be influenced by using the atomic resolution of a scanning tunnelling microscope (STM).
Conventional microscopes use light and lenses to magnify specimens, allowing us to view them with the naked eye or a camera. However, when it comes to atoms and molecules, which are smaller than even the shortest wavelengths of visible light, traditional methods fall short.
To explore these tiny realms, scientists turn to a scanning tunnelling microscope, which operates much like a record player.
With a tip that can be as fine as a single atom, scanning tunnelling microscopes move across a material's surface, measuring properties such as electric current to map each point. However, rather than pressing the tip into the surface like a record player needle, the tip hovers just a single atom’s width above it.
When connected to a power source, electrons travel down the tip and make a quantum leap across the atom-sized gap. The closer the tip is to the surface, the stronger the current; the farther away it is, the weaker the current. This well-defined relationship between tip distance and current allows the microscope to measure and map the surface of the atom or molecule based on the electric current strength. As the tip sweeps across the surface, it builds a precise, line-by-line image of the surface, revealing details invisible to conventional light microscopes.
Single-molecule reactions
Using the atomic precision of a scanning tunnelling microscope, scientists can go beyond mapping the surface of a molecule – they can both reposition single atoms and molecules, and influence and measure the likelihood of specific reaction pathways in individual molecules.
Explaining, Dr Kristina Rusimova, who led the study, said: “Typically, STM technology is employed to reposition individual atoms and molecules, enabling targeted chemical interactions, yet the ability to direct reactions with competing outcomes remained a challenge. These different outcomes happen with certain probabilities governed by quantum mechanics – rather like rolling a molecular die.
“Our latest research demonstrates that STM can control the probability of reaction outcomes by selectively manipulating charge states and specific resonances through targeted energy injection.”
Dr Peter Sloan, senior lecturer in the Department of Physics and co-author of the study, said: “We used the STM tip to inject electrons into toluene molecules, prompting the breaking of chemical bonds and either a shift to a nearby site, or desorption.
“We found that the ratio of these two outcomes was controlled by the energy of the electrons injected. This energy dependence allowed us to achieve control over the probability of each reaction outcome through the targeted "heating" of an intermediate molecular state, guided by precise energy thresholds and molecular barriers.”
PhD student Pieter Keenan, first-author on the research publication, said: “The key here was to maintain identical initial conditions for the test reactions—matching the precise injection site and excitation state – and then vary outcomes based solely on the energy of the injected electrons.
“Within a single molecule’s response to the energy input, the differing reaction barriers drive the reaction outcome probabilities. Altering only the energy input allows us, with high precision, to make a reaction outcome more likely than another – in this way we can ‘load the molecular dice’.”
Professor Tillmann Klamroth from Potsdam University in Germany, added: “This study combines advanced theoretical modelling with experimental precision, leading to a pioneering understanding of the reactions’ probabilities based on the molecular energy landscape. This paves the way for further advances in nanotechnology.”
Looking ahead, Dr Rusimova said: “With applications in both basic and applied science, this advancement represents a major step toward fully programmable molecular systems. We expect techniques such as this to unlock new frontiers in molecular manufacturing, opening doors to innovations in medicine, clean energy, and beyond.”