High-efficiency water splitting process revealed
Reaction of high interest to clean-energy innovations is closely analysed in X-ray study
Experiments at DESY’s light sources PETRA III and FLASH have revealed the complex mode of action behind the artificial splitting of water at its most efficient level. Using X-rays, a team led by DESY leading scientist Simone Techert managed to observe the interaction of water with a perovskite, a class of mineral that is of interest in many new materials and that is well-known as a catalyst for the breaking apart of Water molecules into constituent hydrogen and oxygen molecules. This observation and analysis can help further improve the process, which can be used for efficient and clean production of so-called “green” hydrogen and oxygen for fuel cells and improved battery technologies, among other potential uses, such as in the chemical industry. The research has been published in the journal Accounts of Chemical Research.
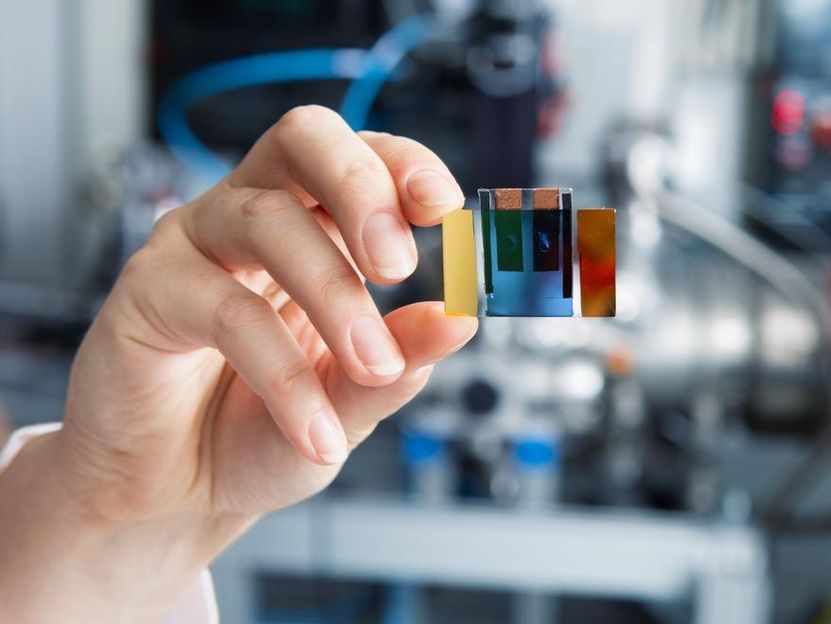
The perovskite LSMO, seen here from another experiment embedded in a solar cell, can catalyse water-splitting at high efficiencies. This helps with the development of so-called 'green hydrogen' and 'green oxygen'. The research team used X-ray spectroscopy to observe the interaction between LSMO and water to better understand how to further make it efficient.
DESY, Gesine Born
Splitting water into hydrogen and oxygen gas is a difficult task. In particular, the formation of the oxygen molecules requires large amounts of energy, since their development requires more intermediate steps than hydrogen’s does. Electrolysis, the most common method of splitting of water molecules using an electrode, has always had the downside of requiring comparably vast amounts of energy. Oxygen hangs onto the hydrogen in water very strongly and because of its reactivity tends to form oxide intermediates that absorb much of that energy before being released as a gas. In the cells of plants and other organisms that undergo photosynthesis, large and complicated systems in the cell are devoted to the breaking down of water molecules in the process of building glucose molecules (instead of hydrogen as a fuel). The water-splitting components in biological systems are the most efficient known, although their exact functions are difficult to decipher. These are of interest for energy storage, in this case the production of green hydrogen and also oxygen, which uses little to no polluting processes.
A class of cube-shaped minerals called perovskites have been noted for their ability to bring down dramatically the energy of electrolysis under ambient conditions. “The perovskites we use deviate in their catalytic activity from what is expected,” Techert says. “We wanted to find out exactly why they are as efficient as they are”.
Techert’s team used soft X-ray spectroscopy to monitor the reaction as it takes place. Their perovskite of interest is itself artificially generated: Called LSMO, it contains atoms of lanthanum and strontium in a manganese oxide crystal structure.
“Perovskites are very useful because it’s relatively easy to systematically replace the functional atoms within them,” says Marcel Risch, an expert on perovskites and their use in the production of green hydrogen at Helmholtz-Zentrum Berlin.
The team used a special reaction chamber to monitor the motion of electrons between the water molecules and the perovskite during an electrolysis reaction. The chamber, called ChemRIXS, was developed at DESY for free-electron lasers like FLASH but can also interface with synchrotron-based light sources such as PETRA III. The team used both facilities to collect their data. With ChemRIXS, researchers can combine complex chemical environments with a technique called resonant inelastic soft-X-ray scattering that reveals the motion of electrons – the fundament of chemical reactions.
In this particular electrolysis reaction catalysed by the LSMO perovskite, the team was able to follow the reaction on the surface of the catalyst. The results showed that the manganese in LSMO is kept at a very high positive charge by the other atoms in the crystal, allowing it to attract the oxygen away from the hydrogens in the water molecule during the reaction. This enabled the two electrodes used in the electrolysis to produce both hydrogen and oxygen with far less energy. Instead of forming oxides that absorb large amounts of reaction energy, the oxygen is attracted to manganese in the LSMO. The manganese changes its attractiveness in the course of the reaction, enabling the oxygen to bind to itself and form a gas at a lower energy than if oxides had formed.
“Because of its material properties, LSMO is one of the favoured electrode materials used to produce oxygen in the water splitting process,” Techert says. “The oxygen production is a four-step reaction, which is tricky to realise when aiming for low-energy consumption for this reaction. In the experiments, we could watch the intermediates formed on the LSMO surface with water before the oxygen is formed – that knowledge can help make an already efficient process even more so if we can consider certain defined material changes.”
To work on the efficiency of the oxygen process, the team dissolved potassium hydroxide in the water. Beyond providing more real-world conditions in the lab, the team found that the more alkaline water helps the electrolysis reaction progress more efficiently by providing free ions.
“This is pioneer research, where we are seeing what is at all possible,” says Risch. The team will examine in future experiments with the same methods the dynamics of the hydrogen and oxygen processes.
The research included scientists from DESY, the University of Göttingen, and Helmholtz-Zentrum Berlin in Germany, and the Paul Scherrer Institute and the company XRnanotech in Switzerland.