A Window into the Nanoworld: Scientists Develop New Technique to Image Fluctuations in Materials
New opportunities for materials research
A team of scientists, led by researchers from the Max Born Institute in Berlin and Helmholtz-Zentrum Berlin in Germany and from Brookhaven National Laboratory and the Massachusetts Institute of Technology in the United States has developed a revolutionary new method for capturing high-resolution images of fluctuations in materials at the nanoscale using powerful X-ray sources. The technique, which they call Coherent Correlation Imaging (CCI), allows for the creation of sharp, detailed movies without damaging the sample by excessive radiation. By using an algorithm to detect patterns in underexposed images, CCI opens paths to previously inaccessible information. The team demonstrated CCI on samples made of thin magnetic layers, and their results have been published in Nature.
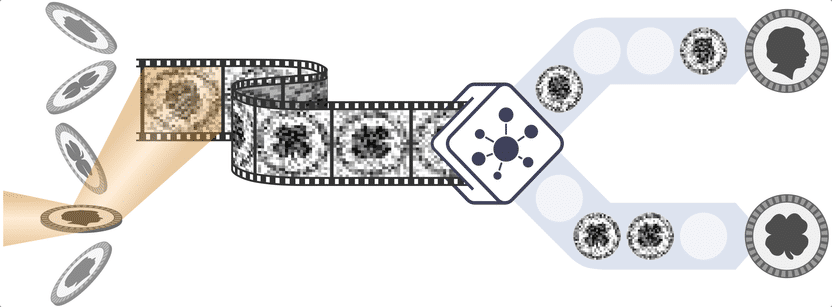
The principle of Coherent Correlation Imaging is used to image a random process, such as, figuratively, a coin toss. A single, brief image of the coin may not be sufficiently exposed to clearly identify the image on the coin. However, a new algorithm can sort and combine multiple images to produce clear images of both sides of the coin, which can then be accurately assigned to the moment of exposure.
Christopher Klose / MBI
The microscopic realm of the world is constantly in motion and marked by unceasing alteration. Even in seemingly unchanging solid materials, these fluctuations can give rise to unusual properties; one example being the lossless transmission of electrical current in high-temperature superconductors. Fluctuations are particularly pronounced during phase transitions, where a material changes its state, such as from solid to liquid during melting. Scientists also investigate very different phase transitions, such as from non-conductive to conductive, non-magnetic to magnetic, and changes in crystal structure. Many of these processes are utilized in technology, and also play a crucial role in the functioning of living organisms.
The problem: Too much illumination might damage the sample
Studying these processes in detail, however, is a difficult task, and capturing a movie of these fluctuation patterns is even more challenging. This is because the fluctuations happen quickly and take place at the nanometer scale – a millionth of a millimeter. Even the most advanced high-resolution X-ray and electron microscopes are unable to capture this rapid, random motion. The problem is fundamentally rooted, as exemplified by this principle of photography: in order to capture a clear image of an object, a certain level of illumination is required. To magnify the object, that is to “zoom in”, more illumination is needed. Even more light is necessary when attempting to capture a fast motion with a short exposure time. Ultimately, increasing the resolution and decreasing the exposure time leads to a point where the object would be damaged or even destroyed by the illumination required. This is exactly the point science has reached in recent years: snapshots taken with free-electron lasers, the most intense X-ray sources available today, inevitably led to the destruction of the sample under study. As a result, capturing a movie of these random processes consisting of multiple images has been deemed impossible.
New approach: using an algorithm to detect patterns in dimly lit pictures
An international team of scientists has now found a solution to this problem. The key to their solution was the realization that the fluctuation patterns in materials are often not entirely random. By focusing on a small portion of the sample, the researchers observed that certain spatial patterns repeatedly emerged, but the exact timing and frequency of these patterns were unpredictable.
The scientists have developed a novel non-destructive imaging method called Coherent Correlation Imaging (CCI). To create a movie, they take multiple snapshots of the sample in quick succession while reducing the illumination enough to keep the sample intact. However, this results in individual images where the fluctuation pattern in the sample becomes indistinct. Nevertheless, the images still contain sufficient information to separate them into groups. To accomplish this, the team first had to create a new algorithm that analyzes the correlations between the images, hence the method’s name. The snapshots within each group are very similar and thus likely to originate from the same specific fluctuation pattern. It is only when all shots in a group are viewed together that a clear image of the sample emerges. The scientists are now able to rewind the film and associate each snapshot with a clear image of the sample’s state at that moment in time.
An example: Filming the „dance of domains” in magnetic layers
The scientists created this new method to tackle a specific problem in the field of magnetism: microscopic patterns that occur in thin ferromagnetic layers. These layers are divided into regions known as domains, in which the magnetization points either upward or downward. Similar magnetic films are used in modern hard drives where the two different types of domains encode bits with “0” or “1”. Until now, it was believed that these patterns were extremely stable. But is this really true?
To answer this question, the team investigated a sample consisting of such a magnetic layer at the National Synchrotron Light Source II on Long Island near New York City, using the newly developed CCI method. Indeed, the patterns remained unchanged at room temperature. But at a slightly elevated temperature of 37°C (98°F), the domains began to move back and forth erratically, displacing each other. The scientists observed this “dance of the domains” for several hours. Subsequently, they created a map showing the preferred location of the boundaries between the domains. This map and the movie of the movements led to a better understanding of the magnetic interactions in the materials, promoting future applications in advanced computer architectures.
New opportunities for materials research at X-ray sources
The scientists’ next objective is to employ the novel imaging method on free-electron lasers, such as the European XFEL in Hamburg, to gain deeper insights into even faster processes at the smallest length scales. They are confident that this method will improve our understanding of the role of fluctuations and stochastic processes in the properties of modern materials, and as a result, discover new methods of utilizing them in a more directed manner.
Original publication
Christopher Klose, Felix Büttner, Wen Hu, Claudio Mazzoli, Kai Litzius, Riccardo Battistelli, Ivan Lemesh, Jason M. Bartell, Mantao Huang, Christian M. Günther, Michael Schneider, Andi Barbour, Stuart B. Wilkins, Geoffrey S. D. Beach, Stefan Eisebitt and Bastian Pfau; Coherent correlation imaging for resolving fluctuating states of matter; Nature (online) (2023)