Why optical cavities curb the rate of chemical reactions
“This works puts the controversial field of polaritonic chemistry onto a different level”
Chemical processes are all around us. From novel materials to more effective medicines or plastic products – chemical reactions play a key role in the design of the things we use every day. Scientists constantly search for better ways to control these reactions, for example to develop new materials. Now an international research team led by the MPSD has found an explanation why chemical reactions are slowed down inside mirrored cavities, where molecules are forced to interact with light. Its work, published in Nature Communications, is a key step in understanding this experimentally observed process.
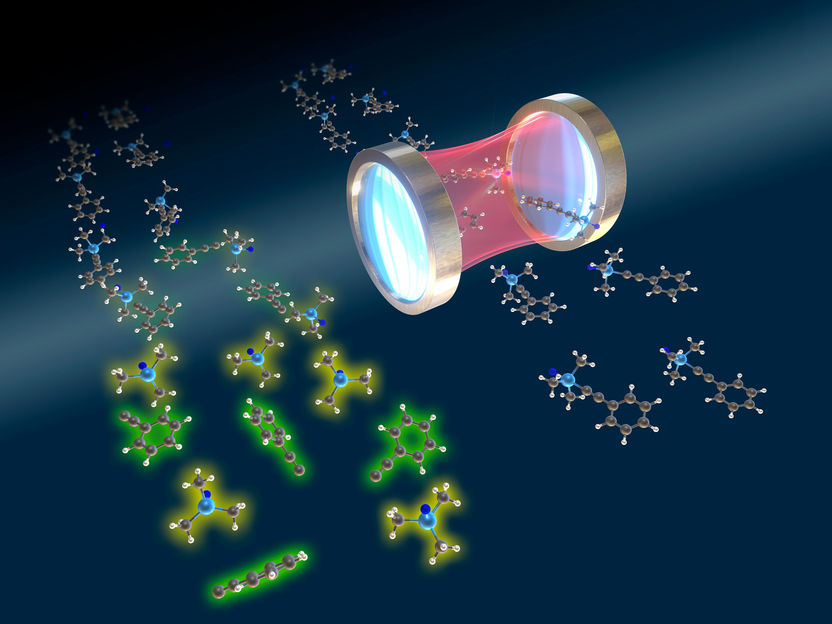
Resonant vibrational strong-coupling can inhibit chemical reactions. Strong resonant coupling between cavity and vibrational modes can selectively inhibit a chemical reaction.
E. Ronca / C. Schäfer
Chemical reactions occur on the scale of atomic vibrations - one million times smaller than the thickness of a human hair. These tiny movements are difficult to control. Established methods include the control of temperature or providing surfaces and complexes in solution made from rare materials. They tackle the problem on a larger scale and cannot target specific parts of the molecule. Ideally, researchers would like to provide only a small amount of energy to some atoms at the right time, just like a billiard player wants to nudge just one ball on the table.
In recent years, it became clear that molecules undergo fundamental changes when they are placed in optical cavities with opposing mirrors. Inside those confines, the system is forced to interact with virtual light, or photons. Crucially, this interaction changes the rate of chemical reactions – an effect that was observed in experiments but whose underlying mechanism remained a mystery.
Now a team of theoretical physicists from Germany, Sweden, Italy and the U.S.A. has come up with a possible explanation, which qualitatively agrees with the experimental results. The team involved researchers from the Max Planck Institute for the Structure and Dynamics of Matter (MPSD) in Hamburg, Germany, Chalmers University of Technology in Sweden, the Center for Computational Quantum Physics at the Flatiron Institute, Harvard University (both in the U.S.A.), and the Istituto per i Processi Chimico Fisici at the CNR (National Research Council) in Italy.
Using an advanced theoretical method, called Quantum-Electrodynamical Density-Functional Theory (QEDFT), the authors have unveiled the microscopic mechanism which reduces the chemical reaction rate, for the specific case of the deprotection reaction of 1-phenyl-2-trimethylsilylacetylene. Their findings are in agreement with the observations by the group of Thomas Ebbesen in Strasbourg.
The team discovered that the conditions inside the optical cavity affect the energy which makes the atoms vibrate around the molecule’s single bonds, which are critical for the chemical reaction.
Outside the cavity, that energy is usually deposited in a single bond during the reaction, which can ultimately break the bond – a key step in a chemical reaction. “However, we find that the cavity introduces a new pathway, so that the energy is less likely to be funneled only into a single bond,” says lead author Christian Schäfer. “This is the key process which inhibits the chemical reaction, because the probability to break a specific bond is diminished.”
Manipulating materials through the use of cavities (the so-called ‘polaritonic chemistry‘) is a powerful tool with many potential applications, according to the paper’s author Enrico Ronca, who works at CNR: “For instance, it was observed that coupling to specific vibrational excitations can inhibit, steer, and even catalyze a chemical process at room temperature. Our theoretical work enhances the understanding of the underlying microscopic mechanisms for the specific case of a reaction inhibited by the field.”
While the authors point out that important aspects remain to be understood and further experimental validation is required, they also highlight the special role of this new direction. “This works puts the controversial field of polaritonic chemistry onto a different level,” adds Angel Rubio, the Director of the MPSD’s Theory Department. “It provides fundamental insights into the microscopic mechanisms that enable the control of chemical reactions. We expect the present findings to be applicable to a larger set of relevant reactions (including click chemical reactions linked to this year’s Nobel Prize in chemistry) under strong light-matter coupling conditions.“