How to program materials
Predict the properties of composite materials
Can the properties of composite materials be predicted? Empa scientists have mastered this feat and thus can help achieve research objectives faster. This leads, for instance, to better recycling techniques and electrically conductive synthetic materials for the solar industry.
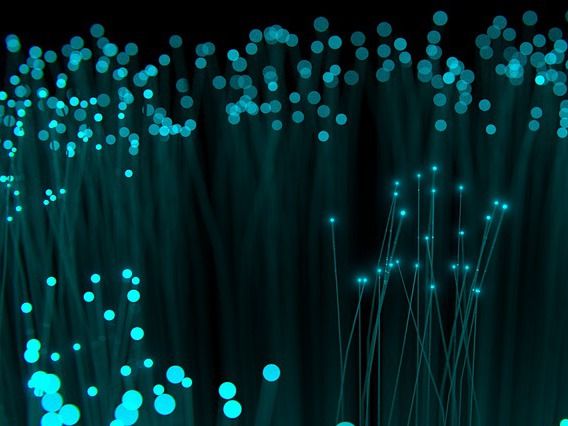
Fibers are a matter of experience. The properties can be calculated (symbol image).
JKimsey, pixabay.com, CC0
Ali Gooneie simulates on his computer what holds the world together right at its very core: atoms, molecules, molecular chains and bundles – then lumps and fibers, which emerge thereof. With his calculations, the Empa researcher can also explain properties we can feel with our fingertips: smooth and rough surfaces, flexible and rigid materials, heat-conductive substances and insulators.
Many of these properties have their origin deep inside the materials. Metal or wood, plastic or ceramics, stone or gel – all of these have been examined many times before. However, what about composite materials? How do the properties of such materials come about and how can they be altered in a desired way? A tedious trial-and-error approach in the lab is no longer sufficient in today’s fast-paced research; nowadays, you need computer-assisted predictions to be able to decide quickly which experimental path you will have to take.
Gooneie is one of many computer simulation experts who work in various research labs at Empa. He studied polymer technology at Amirkabir University of Technology in Tehran and did his doctorate at the University of Leoben in Austria. “Although after my engineering degree I immersed myself ever deeper in the world of physics formulae, I never lost touch with the real world,” he says. “For me, simulations are not an end in themselves. I use them to explain the effects we observe in materials.”
What does a hair feel like? And above all: why?
In order to understand what exactly Gooneie is calculating, it is worth considering a biological polymer composite fiber material we all know very well: hair. Freshly washed, it feels soft and flexible. When it is dry, it crackles like electricity; and when wet, it squeaks like rubber. We can cut it, pull it out, singe it, perm it, bleach it and blow-dry it. But where do all these properties come from?
Hair consists of individual amino acids, which combine to form long-chain proteins known as keratins. These long keratin molecules bond to form threads and fiber bundles. A complex made of cell membranes cements these fiber bundles together. These fiber bundles are encased by several layers of dead horn scales lying staggered on top of each other like the scales of a pinecone. Therefore, the properties of hair would be inexplicable if only the basic chemical building blocks – the amino acids – were considered. Understanding the overarching structure is crucial.
So let us, in our minds, zoom out of the chemical structure and see the molecules only as globules, which are connected like on a pearl necklace. Now the picture is no longer determined by chemistry, but by the collisions and friction effects of these pearl chains. Experts use coarse mathematical models for their calculations.
Eventually, we arrive in a dimension that we can see and feel: the millimeter range, where hair is considered a homogenous material – the fine structure is no longer important. The material's macroscopic properties can be described and predicted using the “finite element method”.
Detailed understanding of fibers
Until only a few years ago, there was no such multidimensional approach in the polymer composites sector. With his research at the University of Leoben, Ali Gooneie had refined this approach, which made him a perfect fit for Empa. The simulation expert moved to St. Gallen and is now conducting research in Empa's Advance Fibers lab under Manfred Heuberger.
One of Heuberger’s research goals is to refine synthetic fibers – an economically important topic: These days, around two thirds of all fibers used worldwide are produced synthetically. A synthetic fiber is considerably more than a fine plastic filament. They only become “fibers” if their molecular structure comprising small crystals and aligned molecules is geared towards the desired properties – such as flexibility or firmness. Only if the fiber structure is known from the nanometer to the micrometer scale can the properties of the product be set specifically during processing.
Conductive polymer composites
Gooneie has already overseen several projects. For instance, one was aimed at embedding carbon nanotubes (CNT) in a polyamide matrix. At the right dosage, CNTs can give a synthetic material electric conductivity – which makes this material interesting for the photovoltaics industry, for example. But what is the perfect amount of nanotubes to be mixed in? Should the tubes be the same length or would a mixture of lengths provide better results?
So far, it has been common for composite researchers to narrow down and solve the problem at hand with a series of experiments. Ali Gooneie, however, tackles the problem from a theoretical angle and uses his multi-dimensional simulation methods. The solution he came up with: A mixture of CNT with different lengths yields electrical conductivity the fastest. Ultimately, he succeeded in predicting the way, in which the nanotubes are arranged in the polymer – irrespective of the speed, with which the processing takes place.
At the same time the calculations were carried out, the researchers got their first experiment going: In a hot extruder at 245 degrees Celsius, they mixed nanotubes in various proportions into the polyamide matrix. It turned out that an admixture of 0.15 percent by weight yielded the best results in terms of electrical conductivity. Hand in hand with lab experiments, applied mathematics provided an elegant solution to the problem.
Gentle PET recycling
Simulation calculations can also achieve a lot in recycling projects. The Swiss collected almost 48,000 tons of PET bottles in 2018. From this, industry gained 35,000 tons of recycled PET. The synthetic material is highly sought-after as it is mechanically resilient, air and gas-tight, and can withstand high temperatures. However, PET cannot be recycled an unlimited number of times. If the material is remelted too often, chemical reactions take place within the material: The molecules oxidize, cross-link and form lumps, and the material becomes viscous and translucent.
An additive called DOPO-PEPA could change all this. In fact, the material is a flame retardant developed by Empa researcher Sabyasachi Gaan, also in the Advance Fibers lab. Now the researchers want to explore whether it can also serve as a lubricant and preservative for PET recycling. Gooneie began by estimating whether DOPO-PEPA can be mixed into PET at the intended temperature at all. Then he calculated how the pearl necklace of PET molecules would move in the melt, how the DOPO-PEPA molecules would squeeze between them, and when an equilibrium would appear in the mixture.
The result: An admixture of a few percent of DOPO-PEPA is already sufficient to allow recycled PET to flow well. Thanks to higher mathematics at Empa, recycling will soon run much more smoothly.