A crystal method
Unlocking another piece of the puzzle that is crystal growth
From Mother Nature to our must-have devices, we're surrounded by Crystals. Those courtesy of the former, such as ice and snow, can form spontaneously and symmetrically. But the silicon-based or gallium nitride crystals found in LEDs and other electronics require a bit of coaxing to attain their ideal shapes and alignments.
At UC Santa Barbara, researchers have now unlocked another piece of the theoretical puzzle that governs the growth of crystals -- a development that may save time and energy in the many processes that require crystal formation.
"The way most industrial processes are designed today is by doing an exhaustively large number of experiments to find out how crystals grow and at what rate they grow under different conditions," said UCSB chemical engineer Michael Doherty. Snowflakes, for instance, form differently as they fall, depending on variable conditions such as temperature and humidity, hence the widely held belief that no two are alike. After determining the optimal conditions for the growth of the crystal of choice, Doherty added equipment must be designed and calibrated to provide a consistent growing environment.
However, by pooling decades of expertise, Doherty, along with UCSB colleague Baron Peters and former graduate student Mark Joswiak (now at Dow Chemical) have developed a computational method to help predict growth rates for ionic crystals under different circumstances. Using a relatively simple crystal -- sodium chloride (NaCl, more familiarly known as table salt) -- in water, the researchers laid the groundwork for the analysis of more complex crystals.
Ionic crystals may appear to the naked eye -- and even under some magnification -- to consist of perfectly smooth and even faces. But look more closely and you'll often find they actually contain surface features that influence their ability to grow, and the larger shapes that they take.
"There are dislocations and around the dislocations there are spirals, and around the spirals there are edges, and around the edges there are kinks," Peters said, "and every level requires a theory to describe the number of those features and the rates at which they change." At the smallest scale, ions in solution cannot readily attach to the growing crystal because water molecules that solvate (interact with) the ions are not readily dislodged, he said. With so many processes occurring at so many scales, it's easy to see how difficult it can be to predict a crystal's growth.
"The largest challenge was applying the various techniques and methods to a new problem -- examining ion attachment and detachment at surface kink sites, where there is a lack of symmetry coupled with strong ion-water interactions," Joswiak said. "However, as we encountered problems and found solutions, we gained additional insight on the processes, the role of water molecules and differences between sodium and chloride ions."
Among their insights: Ion size matters. The researchers found that due to its size, the larger chloride ion (Cl-) prevents water from accessing kink sites during detachment, limiting the overall rate of sodium chloride dissolution in water.
"You have to find a special coordinate system that can reveal those special solvent rearrangements that create an opening for the ion to slip through the solvent cage and lock onto the kink site," Peters said. "We demonstrated that at least for sodium chloride we can finally give a concrete answer."
This proof-of-concept development is the result of the Doherty Group's expertise with crystallization processes coupled with the Peters Group's expertise in "rare events" -- relatively infrequent and short-lived but highly significant phenomena (such as reactions) that fundamentally change the state of the system. Using a method called transition path sampling, the researchers were able to understand the events leading up to the transition state. The strategy and mechanistic insights from the work on sodium chloride provides a blueprint for predicting growth rates in materials synthesis, pharmaceuticals and biomineralization.
Original publication
Other news from the department science
Most read news
More news from our other portals
See the theme worlds for related content
Topic world Synthesis
Chemical synthesis is at the heart of modern chemistry and enables the targeted production of molecules with specific properties. By combining starting materials in defined reaction conditions, chemists can create a wide range of compounds, from simple molecules to complex active ingredients.
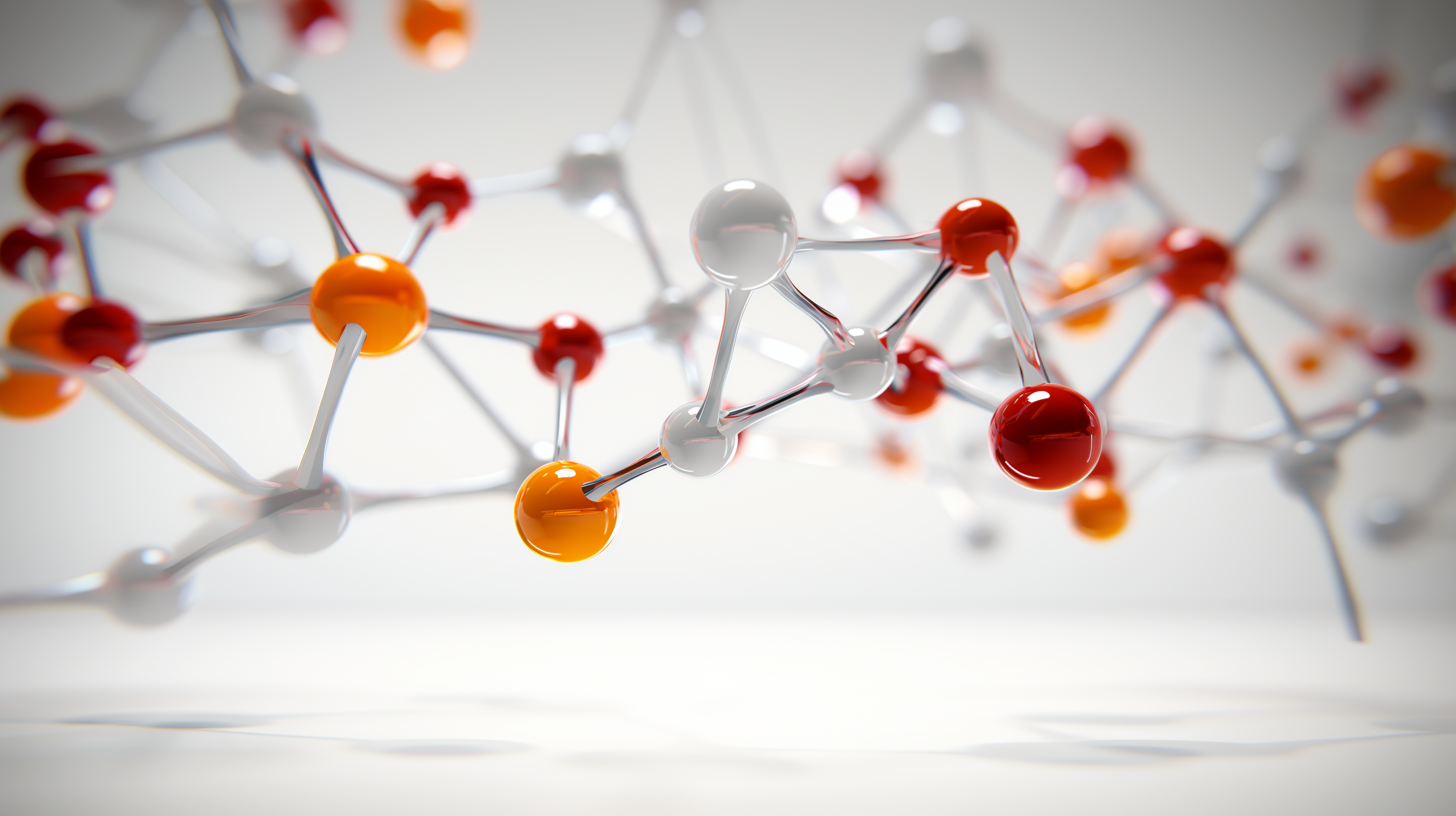
Topic world Synthesis
Chemical synthesis is at the heart of modern chemistry and enables the targeted production of molecules with specific properties. By combining starting materials in defined reaction conditions, chemists can create a wide range of compounds, from simple molecules to complex active ingredients.